Omega-3 LCPUFA Intake-Induced Molecular Changes in Type 2 Diabetes
This article at a glance
development of a reduced ability to secrete insulin. Chronic resting hyperglycemia and increased post-prandial hyperglycemic responses that ensue from inadequate blood sugar control lead to vascular damage over time. Retinopathy, kidney failure, neuropathy, and poor wound healing responses are characteristic sequelae of the microvascular complications in type 2 diabetics. Macrovascular complications include coronary artery disease, cerebrovascular disease and peripheral artery disease. A majority of type 2 diabetics is obese, but the disorder can also appear during normal aging. In addition to improved focus on prevention as a key factor limiting the increase in type 2 diabetes prevalence, the identification of affordable approaches to reduce the increased risk of microvascular complications in current diabetics is also important. Lifestyle and nutritional changes, including increased intermittent physical activity and intake of foods with a lower glycemic index and load, are beneficial in order to recover normal levels of insulin sensitivity, blood glucose, and body weight. It is less clear though if the vascular injury and increased mortality from cardiovascular causes in type 2 diabetics are modifiable by achieving normoglycemia and a more active lifestyle alone. Aggressive control of glycaemia, blood pressure and LDL-cholesterol that achieve a very marked (>10%) decrease in body weight and a near-normal glycated hemoglobin level is associated with a reduced risk of death from cardiovascular causes and infarction. Hyperglycemia in diabetes imparts a long-term increased risk of microvascular complications and cardiovascular disease, a process termed “glycemic memory,” which is likely mediated by epigenetic changes. Demonstrated proof of efficacy of all the modifiable factors that may improve the short and long-term health of the large global population of type 2 diabetics is therefore of interest. Adequate omega-3 long-chain polyunsaturated fatty acids (omega-3 LCPUFA) intake and tissue levels can reduce some of the risk factors associated with cardiometabolic disease, including vascular patency and blood pressure, immune cell activation in vascular inflammation, and hypertriglyceridemia. Of particular interest to type 2 diabetes is the ability of omega-3 LCUFA intake to reduce VLDL-associated hypertriglyceridemia, which may help reduce ectopic fat accumulation in the pancreas and liver, and may contribute to diabetes reversal in patients submitted to a low calorie intake regime. Although the modulatory effects of omega-3 LCPUFA intake on lipid homeostasis and inflammation are relatively well recognized, their effects on glucose homeostasis are considered to be more complex and are still poorly understood. Adequate tissue levels of omega-3 LCPUFA may contribute to normal insulin resistance, as suggested by preclinical research and observational studies. However, intervention studies in humans that have addressed the effect of increased omega-3 LCPUFA intake on insulin resistance have provided mixed results. Large systematic reviews have not found convincing evidence for an effect of omega-3 LCPUFA intake on glycemic control in type 2 diabetics. To better understand the reasons for the varied effects of omega-3 LCPUFA intake and tissue status on glucose metabolism in type 2 diabetics, more detailed studies on the dysfunctional molecular responses in this disease and their potential modulation by omega-3 LCPUFA may provide new insight. Two recent studies have made such an assessment, and used samples from two double-blind randomized controlled trials. Both studies compared the effects of dietary supplementation with EPA/DHA-rich fish oil, flaxseed oil (rich in alpha-linolenic acid) and a vegetable oil that was low in omega-3 PUFA content (soybean oil and corn oil).
The first study was carried out by Karakas and colleagues from the Department of Internal Medicine, and the Genome Center at the University of California at Davis, CA, and the Department of Veteran Affairs Northern California Health Care System, Mather, CA, USA. The study focused on US American women (20-45 years old) with polycystic ovary syndrome, a disorder characterized by insulin resistance. Fifty-four women, divided into three intervention groups, received 3.5 g a day of EPA/DHA from fish oil, alpha-linolenic acid from flaxseed oil, or an equivalent volume of encapsulated soybean oil, for six weeks. Metabolites in plasma were measured by gas chromatography-mass spectrometric (GC-MS) analysis at the beginning and the end of the intervention period. An extraction and derivatization step was employed, which allowed relative quantification (so-called enrichment analysis) of mostly polar metabolites, which were identified by comparison of the retention index and mass spectral similarity with a database of >1000 metabolites. In addition, various parameters of glucose homeostasis and cardiovascular disease risk were measured. After six weeks, women that had received fish oil had an improved overall insulin secretion (insulin response) than the other groups, which showed no change compared to baseline. Women receiving fish oil had a small but significant decrease in hemoglobin A1c (HbA1c), a glycated form of hemoglobin, and a marker of long-term plasma glucose levels. HbA1c refers to the stable ketoamine formed after intermolecular rearrangement (an Amadori rearrangement) of the reaction product formed between glucose and the amino-terminal valine group of hemoglobin (HbA0). Such ketoamines formed from the reaction of glucose with reactive amine groups present in a range of cellular proteins, including hemoglobin and also specific lipids, can, under conditions of oxidative stress, further react to form so-called “advanced glycation end-products” (AGE’s). These substances signal tissue damage and potently activate inflammatory responses. A chronically elevated rate of AGE formation, such as that occurring in diabetes, is believed to be causally involved in vascular tissue damage. Baseline levels of HbA1c were at the high end of the normal range in this study. The intake of fish oil worsened the Matsuda insulin sensitivity index, a measure of the efficacy of glucose removal from the circulation after a glucose challenge. Fish oil supplementation decreased early insulin release (upon an intravenous glucose challenge) as did soybean oil intake, but increased overall insulin levels in blood upon an oral glucose challenge compared to soybean oil intake. Flaxseed oil intake did not affect insulin release. As expected, fish oil supplementation lowered triglyceride levels, an effect shared with flaxseed oil intake. Fish oil and flaxseed oil intake had no significant effect on the Disposition Index, a measure of β-cell function and compensatory insulin release, whereas soybean oil intake reduced this parameter. There was no effect on glucose levels for any of the interventions tested. Taken together, EPA/DHA intake during six weeks slightly reduced hemoglobin glycation and further ameliorated insulin sensitivity, but increased insulin blood levels, perhaps as a result of a slower breakdown of insulin, since pancreatic function was unaffected. These intricate modulatory effects of fish oil intake on glucose homeostasis were accompanied by several significant changes in metabolite abundance, particularly amino acids. The major changes observed were: increased serum levels of branched chain amino acids (BCAAs) (isoleucine, leucine and valine), decreased levels of cysteine and its oxidized disulfide form cystine, and significant increases in several other amino acids (glycine, serine, glutamic acid, glutamine and proline). These changes were not seen after flaxseed oil intake, except for similar but less pronounced changes in cysteine and cystine levels. Soybean oil supplementation increased the levels of isoleucine, valine, methionine, glycine and proline, and had an effect on cysteine and cystine similar to that of flaxseed oil. Using pathway enrichment analysis, a technique that allows the mapping of metabolite changes onto known metabolic pathways, the researchers concluded that fish oil supplementation affected amino acid synthesis and degradation pathways, pathways involved in the metabolism of small organic substances like formaldehyde, the degradation of amines and polyamines, the degradation of purine nucleotides, and the biosynthesis of alanine, glycine and L-carnitine. The impact of soybean oil intake was limited to amino acid biosynthesis and degradation, and flaxseed oil mainly affected alanine biosynthesis and cysteine degradation. Other studies have documented the effect of omega-3 LCPUFA on essential amino acid metabolism. This involves effects on the gene transcription of enzymes regulating BCAA breakdown, forming a link between increased BCAA levels and their known insulin resistance-promoting effect. A biosignature of BCAAs (leucine, isoleucine, and valine) and related amino acids (phenylalanine, tyrosine, methionine, alanine and histidine) consequent to a change in their catabolism, has been proposed as a means to distinguish individuals who are cardiometabolically “unwell” (including displaying insulin resistance) from those who are more metabolically healthy. Insulin resistance may lead to a compensatory release of insulin, a process also known to be promoted by BCAAs and in line with the observations made in this study. In addition to recognizing the impact of fish oil intake on amino acid metabolism, the intake of soybean oil produced a metabolic response that resembled fish oil intake more closely than the intake of ALA-rich flaxseed oil. This observation suggests that the triglyceride-lowering effect of EPA/DHA and plant-derived ALA can be dissociated from the effects of omega-3 LCPUFA (or strictly fish oil as studied here) on amino acid metabolism. The second intervention trial assessed changes in the metabolomic profile of a group of Chinese type 2 diabetic adults after supplemental fish oil intake. The study was performed by Zheng and colleagues from the Department of Food Science and Nutrition, at Zhejiang University, Hangzhou, China, in collaboration with the Huazhong University of Science and Technology, and the Agricultural University, both in Wuhan, China, and the University of Cambridge, UK. The intervention study was carried out in three geographically different clinical centers in China. Metabolomic analysis was performed on serum samples from 53 participants from one of the participating centers. Participants (age 35-80 years, average age ~70 years) were assigned to one of three study groups that during a period of six months received fish oil (2 gram EPA+DHA in 4 capsules/day; n=20), flaxseed oil (2.5 gram ALA in 4 capsules/day; n=20), or corn oil (4 capsules/day; n=19). A group of healthy controls (n=17) without type 2 diabetes was recruited in parallel. Untargeted metabolic profiling was carried out by ultra-performance liquid chromatography interfaced with tandem mass spectrometric analysis, as well as by GC-MS analysis of derivatized samples. In total 407 distinct signals were detected, which were matched against a library of > 3300 compounds. Biochemical parameters (lipoprotein-associated cholesterol, triglycerides, glucose levels in serum, and fatty acids in erythrocyte membrane phospholipids) as well as heart rate, blood pressure and body mass index, were measured at the beginning and end of the intervention period. The multisite intervention trial showed that marine omega-3 LCPUFA supplementation did not improve insulin resistance or fasting glucose and insulin levels. However, HbA1c levels decreased significantly over time when compared to the corn oil group, as well as triglycerides and LDL-cholesterol.
In the EPA/DHA-supplemented diabetic individuals, significant changes were measured in the levels of omega-3 PUFA: increased serum levels of the free fatty acid form of EPA, DHA and docosapentaenoic acid (ω-3), as well as EPA esterified to phosphatidylcholine (the most abundant phospholipid type). Much less expected, the only other metabolite with a significantly changed level (a four-fold increase) was 3-carboxy-4-methyl-5-propyl-2-furopropanoate (abbreviated CMPF; Figure 1). This substance is known as a metabolite of furan fatty acids (or F-acids), a specific class of fatty acids that contain a methylated furan moiety. Furan fatty acids are formed by microscopic organisms (microalgae and bacteria) and bio-accumulate in marine organisms such as fish, possibly in a similar fashion to the trophic transport observed with omega-3 LCPUFA. Levels of F-acids in food vary, but can be significant in fish tissues (~0.1-4 g/100 g), and levels of 0.1 g/100g have been reported in fish oil. The furan moiety confers free radical scavenging activity to this type of fatty acids, but any specific biological roles remain largely unknown. Upon fish consumption, furan fatty acids are absorbed into the circulation, then metabolized to CMPF and excreted via urine. Bacteria in the gastrointestinal tract of fish may generate furan fatty acids directly.
Increased CMPF levels (from a measurable background level) suggest that this reflects an increased intake of furan fatty acids from the fish oil supplement that was given, in line with a previous study that documented the absorption of this poorly documented group of dietary antioxidants. Linear correlations were found between the change in serum EPA or DHA and CMPF, and increased serum CMPF was associated with lower triglyceride levels. Further studies need to be carried out to elucidate the origin of CMPF in omega-3 LCPUFA-supplemented diabetic patients, to understand whether dietary furan fatty acids may induce any metabolic changes, and to contrast their effects with those ascribed to straight-chain fatty acids. This is of interest as the average CMPF level was furthermore reported to be higher in the healthy controls than in the diabetic subjects, suggesting that some level of furan fatty acids may be of benefit to metabolic health and/or that a decrease in furan fatty acid degradation to CMPF occurs in diabetic individuals. Interestingly, in both studies insulin resistance was not improved, and was even worsened, by omega-3 LCPUFA intake. It has been suggested that improvements in insulin sensitivity can be observed only at a high omega-3 index, and that the effect is easily missed in intervention studies if omega-3 levels are still inferior to those required to reach a sufficiently elevated omega-3 index. Glycated hemoglobin levels did decrease in both studies, suggesting that on a background of unchanged glucose levels, the glycation of hemoglobin may be diminished, reflecting a lower propensity of glycation reactions of glucose with proteins, a first step in the formation of AGEs that are involved in vascular damage in diabetes. The results point at the possibility that omega-3 LCPUFA intake may reduce oxidative events involved in vascular tissue damage in the diabetic state. Hyperglycemia-mediated cellular injury involves mitochondrial superoxide anion production, and the transformation of Amadori products into reactive AGEs involves an oxidation step. The results of the American intervention study also suggested that changes in amino acid metabolism do not mediate the triglyceride-lowering effects of fish oil intake, but rather may be associated with a decreased glycation.
The limitations of the studies were the relatively small groups for randomized controlled intervention studies. Nevertheless, for the purpose of discovery, these studies have provided new insights that provide indications for renewed focus on the relevant changes that accompany omega-3 LCPUFA intake in type 2 diabetics. These two studies also indicate that amino acid metabolism may play an important role in carbohydrate metabolism and could be a relevant point of modulation of the insulin resistant state of diabetics. Future intervention studies with higher omega-3 LCPUFA intake levels may provide a clearer picture as to whether insulin resistance is also sensitive to improvement. In addition, furan fatty acids present as minor components in specific foods may have been overlooked to date, and may have a potentially interesting activity in metabolic disorders, therefore are worth exploring in more detail. Karakas SE, Perroud B, Kind T, Palazoglu M, Fiehn O. Changes in plasma metabolites and glucose homeostasis during omega-3 polyunsaturated fatty acid supplementation in women with polycystic ovary syndrome. BBA Clin. 2016;5:179-185. [PubMed] Zheng JS, Lin M, Imamura F, Cai W, Wang L, Feng JP, Ruan Y, Tang J, Wang F, Yang H, Li D. Serum metabolomics profiles in response to n-3 fatty acids in Chinese patients with type 2 diabetes: a double-blind randomised controlled trial. Sci. Rep. 2016;6:29522. [PubMed] Worth Noting Albert BB, Derraik JG, Brennan CM, Biggs JB, Smith GC, Garg ML, Cameron-Smith D, Hofman PL, Cutfield WS. Higher omega-3 index is associated with increased insulin sensitivity and more favourable metabolic profile in middle-aged overweight men. Sci. Rep. 2014;4(6697):1-7. [PubMed] Batch BC, Shah SH, Newgard CB, Turer CB, Haynes C, Bain JR, Muehlbauer M, Patel MJ, Stevens RD, Appel LJ, Newby LK, Svetkey LP. Branched chain amino acids are novel biomarkers for discrimination of metabolic wellness. Metabolism 2013;62(7):961-969. [PubMed] Bierhaus A, Hofmann MA, Ziegler R, Nawroth PP. AGEs and their interaction with AGE-receptors in vascular disease and diabetes mellitus. I. The AGE concept. Cardiovasc. Res. 1998;37(3):586-600. [PubMed] Capel F, Acquaviva C, Pitois E, Laillet B, Rigaudiere JP, Jouve C, Pouyet C, Gladine C, Comte B, Vianey Saban C, Morio B. DHA at nutritional doses restores insulin sensitivity in skeletal muscle by preventing lipotoxicity and inflammation. J. Nutr. Biochem. 2015;26(9):949-959. [PubMed] Duvivier BM, Schaper NC, Hesselink MK, van Kan L, Stienen N, Winkens B, Koster A, Savelberg HH. Breaking sitting with light activities vs structured exercise: a randomised crossover study demonstrating benefits for glycaemic control and insulin sensitivity in type 2 diabetes. Diabetologia 2016;Dec 1. [PubMed] Felig P. Insulin is the mediator of feeding-related thermogenesis: insulin resistance and/or deficiency results in a thermogenic defect which contributes to the pathogenesis of obesity. Clin. Physiol. 1984;4(4):267-273. [PubMed] Gholap NN, Davies MJ, Mostafa SA, Khunti K. Diagnosing type 2 diabetes and identifying high-risk individuals using the new glycated haemoglobin (HbA1c) criteria. Br. J. Gen. Pract. 2013;63(607):e165-167. [PubMed] Giacco F, Brownlee M. Oxidative stress and diabetic complications. Circ. Res. 2010;107(9):1058-1070. [PubMed] Hartweg J, Perera R, Montori V, Dinneen S, Neil HA, Farmer A. Omega-3 polyunsaturated fatty acids (PUFA) for type 2 diabetes mellitus. Cochrane Database Syst. Rev. 2008(1):CD003205. [PubMed] Higgins PJ, Bunn HF. Kinetic analysis of the nonenzymatic glycosylation of hemoglobin. J. Biol. Chem. 1981;256(10):5204-5208. [PubMed] Lalia AZ, Lanza IR. Insulin-sensitizing effects of omega-3 fatty acids: Lost in translation? Nutrients 2016;8(6). [PubMed] Lim EL, Hollingsworth KG, Aribisala BS, Chen MJ, Mathers JC, Taylor R. Reversal of type 2 diabetes: normalisation of beta cell function in association with decreased pancreas and liver triacylglycerol. Diabetologia 2011;54(10):2506-2514. [PubMed] Look Ahead Research Group. Cardiovascular effects of intensive lifestyle intervention in type 2 diabetes. N. Engl. J. Med. 2013;369(2):145-154. Look Ahead Research Group. Association of the magnitude of weight loss and changes in physical fitness with long-term cardiovascular disease outcomes in overweight or obese people with type 2 diabetes: a post-hoc analysis of the Look AHEAD randomised clinical trial. Lancet Diabetes Endocrinol. 2016;4(11):913-921. [PubMed] Polycystic ovary syndrome: https://www.pcos.com/insulin-resistance-a-possible-cause-of-pcos/?nabe=5589080592613376:1&utm_referrer=https%3A%2F%2Fduckduckgo.com%2F Reynolds LJ, Credeur PD, Manrique C, Padilla J, Fadel PJ, Thyfault JP. Obesity, type 2 diabetes, and impaired insulin stimulated blood flow: role of skeletal muscle NO synthase and endothelin-1. J. Appl. Physiol. 2016; Oct. 27. [link] Spiteller G. Furan fatty acids: occurrence, synthesis, and reactions. Are furan fatty acids responsible for the cardioprotective effects of a fish diet? Lipids 2005;40(8):755-771. [PubMed] Stefano GB, Challenger S, Kream RM. Hyperglycemia-associated alterations in cellular signaling and dysregulated mitochondrial bioenergetics in human metabolic disorders. Eur. J. Nutr. 2016;55(8):2339-2345. [PubMed] Tandon N, Ali MK, Narayan KM. Pharmacologic prevention of microvascular and macrovascular complications in diabetes mellitus: implications of the results of recent clinical trials in type 2 diabetes. Am. J. Cardiovasc. Drugs 2012;12(1):7-22. [PubMed] Unwin D, Haslam D, Livesey G. It is the glycaemic response to, not the carbohydrate content of food that matters in diabetes and obesity: The glycaemic index revisited. J. Insulin Resist. 2016;1(1):1-9. [PubMed] Vetter W, Wendlinger C. Furan fatty acids - valuable minor fatty acids in food. Lipid Technol. 2013;25(1):7-10. [link] Wahl HG, Tetschner B, Liebich HM. The effect of dietary fish oil supplementation on the concentration of 3-carboxy-4-methyl-5-propyl-2-furanpropionic acid in human blood and urine. J. Sep. Sci. 1992;15(12):815-818. [link] Zheng JS, Lin M, Fang L, Yu Y, Yuan L, Jin Y, Feng J, Wang L, Yang H, Chen W, Li D, Tang J, Cai W, Shi M, Li Z, Wang F, Li D. Effects of n-3 fatty acid supplements on glycemic traits in Chinese type 2 diabetic patients: A double-blind randomized controlled trial. Mol. Nutr. Food Res. 2016;60(10):2176-2184. [PubMed]
- The effects of omega-3 long-chain polyunsaturated fatty acids (LCPUFA) on deranged glucose homeostasis in type 2 diabetics are incompletely understood.
- Metabolomic analyses of blood samples from two recent randomized controlled clinical trials in American and Chinese adults with type 2 diabetes link omega-3 LCPUFA supplementation to changes in branched-chain amino acids and to furan fatty acid metabolism.
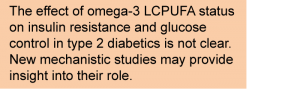
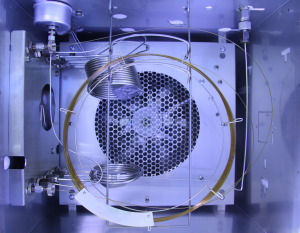
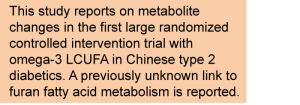
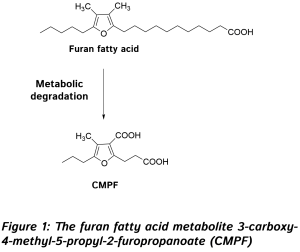
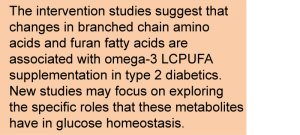